Watershed 101
Minnesota Well Owners Association: https://mnwoo.org
Minnesota Rural Water Association: https://mrwa.com
Thank you to CURE MN for providing these resources. Written by Ariel Herrod, Watershed Sustainability Program Coordinator.
What exactly is a watershed?
​
A body of water’s watershed is the land area that drains surface run-off to that body of water. That is to say, if a drop rain falls on land and the ground is saturated, the water will run downhill until it ends up in a body of water, such as the Minnesota River. In this case, the drop of rain would have fallen within the Minnesota River Watershed: the watershed includes all the land (and other upstream surface water) that funnels rain or snow water to the Minnesota River. Because water runs downhill, topography defines the boundary of a watershed—ridges or high points in a landscape form the line between one watershed and another.
​
It gets a little tricky, though, because watersheds nest inside each other. Look, for example, at the watershed map below. Rain falling in Marshall would run first into the Redwood River, so Marshall is within the Redwood River watershed. However, water in the Redwood River flows eventually into the Minnesota River, so Marshall is also in the Minnesota River watershed. And, because the Minnesota River flows into the Mississippi River, Marshall is in the Mississippi River watershed, too.
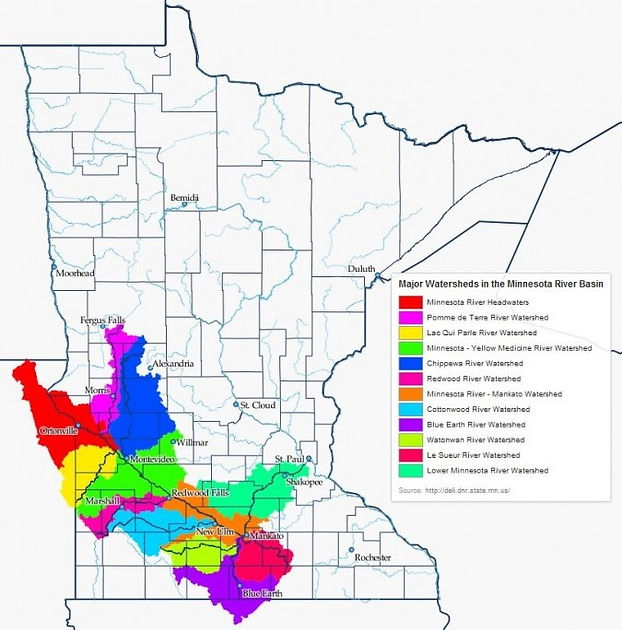
How many watersheds are there?
Yet each of the “watersheds” I named is in some ways arbitrarily defined. Yes, all the land in the Redwood River watershed drains into the Redwood River. But a watershed is really defined by a certain point past which water flows. The DNR (Minnesota Department of Natural Resources) Major Watershed named for the Redwood River really refers to that land area that will drain water through the point of the confluence of the Redwood River and the Minnesota River. If you look at the map of waterways in the United States, you’ll see that there are thousands of waterways, and each point on each waterway has its own watershed, in which the watershed of one point encompasses the watershed of an upstream point. Thus, if we use the
scientific definition of a watershed, there are an infinite number of watersheds in the region, state, and country. I know that infinite watersheds seems overwhelming, but bear with me because others agree and have tried to clarify things.
​
To address the practical uselessness of infinite watersheds, the federal government, through the USGS (United States Geological Survey) and the NRCS (Natural Resources Conservation Service), defined Hydrologic Units based on topographic data. Each of these Hydrologic Units has a code, called the HUC, with the number of digits in the code increasing as the size of the watershed decreases. The relative size of a watershed is often referred to by the length of the HUC. For example, the watershed map above shows all the HUC-8 watersheds in the Minnesota River Watershed.
​
The Minnesota DNR built upon this classification to provide a more detailed look at waters in Minnesota. The MN DNR Major Watershed is equivalent to the federal HUC-8. There are 81 Major Watersheds in Minnesota, and these watersheds are the basis for most administrative watershed work, such as the Watershed Projects or Associations. Furthermore, the Board of Water and Soil Resources’ One Watershed, One Plan program encourages the development of watershed-level plans for each of the 81 Major Watersheds in the state.
​
A Map of Every River in the Contiguous U.S. by Nelson Minar.
While the nomenclature is all very confusing, there is an important lesson to take from all this: you live in a watershed. No ifs, ands, or buts. In Minnesota, rain and snowfall on your home and the land surrounding it, and that water flows downhill and ends up in a body of water somewhere. Which watershed you live in will tell you which body of water your practices on the land affect. Thus, we use watersheds as a geographic unit—a certain defined area—to help us understand why our waterways are in the state that they are in.​
Land Use, Watersheds, and Rivers
This is the key message: what happens on the land is very important for water quality because the path that a drop of water takes from the place where it lands as a raindrop to the river dictates the state of the river. Does it pick up contaminants along the way? Does it move quickly, leading to erosion or flash floods almost immediately after a rainstorm? Does it even travel overland to the river, or does it sink downwards, joining the groundwater reserves underground first? The answers to these questions shape the river and are shaped by the land in the watershed.
In this way, the quality of water in the river is an indicator as much as it is a goal in and of itself. If our rivers are clean and safe for drinking, swimming, and fishing, it means that the watershed is also healthy and functioning as an ecosystem should. That’s why the Minnesota River is the basis for our work, but why the landscape from Ortonville east to Shakopee and just about Fergus Falls south to Blue Earth is where we seek to make change.
Groundwater 101
CURE’s Groundwater 101 series will first introduce you to basic groundwater hydrology; then turn to discuss rural communities’ interactions with groundwater, especially in the context of the highly agricultural Minnesota River watershed; and finally, will look at the role that governmental agencies and policies have in shaping the state of groundwater resources in the state of Minnesota.
​
Groundwater is a big deal. That much is clear. When 75% of Minnesotans turn on the tap, groundwater comes out. About 30% of the world’s freshwater is groundwater. And plentiful, clean water is a key component of Minnesotan identity, even as lake levels drop, wells run dry, and seasonal floods cost the state millions of dollars. Despite that, and increasing press about groundwater resources, there’re still plenty of misconceptions and misunderstandings. However, just because groundwater concepts are tricky to grasp doesn’t mean that you can’t develop a perfectly good working understanding of our state’s underground water resources.
​
Many people think of groundwater as an underground river, and while underground caverns and caves do allow for such formations in some regions of the world, this is rarely the case. So, instead of imagining a river in a cavern, think of a bucket of sand and gravel to which you have added water. The water will sink down into the gaps between the sand particles and gravel, completely saturating a bottom layer in the bucket. Above that wet layer, there will be a layer of damp to dry sand upon which you may place a few tin soldiers for realistic effect—you have just built a pretty decent model of an unconfined or surficial aquifer.
​
Groundwater does move, but very slowly because the water has to squeeze its way through tiny holes and cracks in the rock. And that is where geology becomes extremely important: different rocks have different water holding capacity and allow for different rates of flow, and this results in a huge variety of groundwater resources. There are however, three key, relatively simple factors to consider, and we have quantitative measures for these features.
​
Porosity is the amount of void (air or water) space between particles in a rock or unconsolidated deposit; it is a factor of both the arrangement of rock particles and of the diversity of sizes. Porosity is measured as a percentage—the amount void space as a percent of the total volume of a rock. Specific yield is related to porosity, but it measures how much water will drain from the void spaces in a rock due to gravity. Because of surface tension, some water will cling to the surface of the rock particles, so specific yield is a percentage that is always less than porosity. Specific yield is related to the size of the pores—rocks or unconsolidated deposits with larger pores will have higher specific yields than those with smaller pores because smaller pores result in more particle surface area for the water to cling to.
Permeability, or hydraulic conductivity in groundwater-speak, is related to the porosity of a rock, but it also incorporates the size of and the connections between the pores to indicate how quickly water can actually flow. Hydraulic conductivity is given as a rate, such as millimeters/day.
​
Minnesota’s groundwater provinces
One of the things that makes groundwater so complicated is the fact that invisible underground geology plays a huge role in how groundwater behaves—and in Minnesota, that geology is extremely varied. To reflect this variety, the DNR has divided the state into six groundwater provinces based on a combination of the surficial and bedrock geology for each region of the state.
The bedrock is the base layer, a solid rock that may be exposed to the surface or may be covered by dozens of feet of unconsolidated material such as sand, clay, or glacial till. In Minnesota, there are two main types of bedrock: sedimentary layers of sandstone, limestone, and dolostone in the southeast; and very old, very hard igneous and metamorphic rock in the rest of the state.
​
Above the bedrock are three major categories of surficial deposits: clayey deposits in the western part of the state, sandy deposits in the central region, and very thin or absent surficial deposits that leave the bedrock exposed in the northeast and southeast.
​
When these two maps are overlain, it produces a third map that breaks the state into six different provinces. While the DNR does not use these provinces in any sort of regulatory capacity and acknowledges local variability within provinces, it provides the information with the recommendation that water planners take the unique characteristics of their region into account when evaluating the future productive capacity of ground-water resources.
​
Given this understanding of geology, it becomes easier to interpret the significance of Minnesota’s six groundwater provinces. Provinces 2 and 5, for example, have surficial clay deposits, which means that shallow wells are not likely to yield well. The hard igneous and metamorphic bedrock that underlies provinces 4, 5 and 6 is not very porous, but fractures or cracks in the rock may provide groundwater resources locally. Province 1, where sandy surficial deposits overlay sedimentary bedrock layers that include sandstone, should be a perfect groundwater sweet-spot where both surficial aquifers and deep bedrock aquifers provide plentiful water resources—though the White Bear Lake controversy indicates otherwise. Province 3 is a funny one, with lots of confined aquifers, a feature that occurs as a result of the layer cake sedimentary bedrock.
​
A confined aquifer occurs when a rock or unconsolidated deposit with very low conductivity overlays a permeable aquifer. The confining bed or confining unit that sits on top of the aquifer limits the exchange of water between it and surrounding aquifers. Thus the aquifers are isolated and the groundwater they contain may have very different characteristics, even though they are very close to one another. This makes an intuitive understanding of the local resources difficult to develop because two wells dug right next to each other but reaching to different depths may encounter very different groundwater resources.
​
The Water System
Though “the water cycle” is the common terminology, I have also heard “water system,” which I think better conveys the complexity of the movement of water in our environment. As with all cycles or systems, the starting point is somewhat arbitrary, but for this discussion, let’s start with precipitation. As Minnesota contains (most of) the headwater systems for 3 major watersheds, 99% of the water that enters the state falls from the sky as rain or snow.
Upon reaching the ground, the water may travel one of three ways. Most people clearly understand surface flow because it is visible: we can see water traveling over land as run-off or rivers, lakes, and streams, but this only accounts for about 10-20% of the water that falls as precipitation. The majority of precipitation evapotranspires–that means that it is converted into water vapor either by simple evaporation from puddles, damp ground, or hot sidewalks or by transpiration from plants that absorb and then “exhale” water when they release oxygen. This evapotranspiration generally accounts
for about three-quarters of precipitation.
The remaining water infiltrates the soil surface and then percolates deep into the ground to recharge the aquifers. A USGS estimate indicates that, on average in Minnesota, 20-25% of precipitation recharges the groundwater supplies. While groundwater does eventually flow to surface water bodies, this takes days, weeks, or much longer, dependent in large part on the underground geology.
​
​
​
​
​
Direction of Flow
The image above indicates where recharge and discharge areas may exist on a landscape, with flow lines showing how groundwater moves towards surface water in a discharge area. Why does groundwater flow towards the river? Well, actually it doesn’t always, but let’s start with the example illustrated above. Just like surface water, groundwater movement in an unconfined aquifer is dictated by gravity: many say that it flows “downhill.”
But wouldn’t that just mean flowing straight down towards the center of the earth? Instead I suggest thinking of water in a glass and comparing it to the image above: where’s the contradiction? If you noted that the water table is not flat, while the surface of the water in your glass is, you are right on track.
Just as gravity makes sure that all the water in your glass has moved so that no water stands above the rest, gravity is working to create a level water table. Thus water will flow towards the lowest point in the water table. In groundwater-speak, you would say that groundwater flows towards the point of lowest hydraulic head.
​
Often, the point of lowest hydraulic head is a body of surface water that is whisking away the groundwater, either by evaporation from a lake surface or by movement in a river channel. Because surface water moves so much more quickly than groundwater, the water is rapidly whisked downstream and groundwater continues to flow in that direction. However, in a stable water system, the rate of groundwater recharge is roughly the same as the rate at which water flows to the surface water and downstream, resulting in a steady water table level from year to year.
In some cases, particularly in arid regions where rivers carry water from snow or glacial melt upstream, the river recharges the aquifer, resulting in a cross-section that looks like this:
Regardless of the topographical features, however, the key point is that groundwater flows in the direction of the lowest hydraulic head. Hydrologists determine the hydraulic head at a given point in an aquifer by digging wells (or taking measurements from existing wells): the elevation of the water in the well (above sea level) is the hydraulic head. From there, they can calculate flow direction.
Groundwater Flow in a Confined Aquifer
Understanding the hydraulic head in terms of the water table only works for unconfined or surficial aquifers that do not have a confining bed over them, but never fear—the hydraulic head rule works for confined aquifers as well, and hydrologists perform the measurements in much the same way.
The only tricky part is that because confined aquifers are buried under sometimes 100s of feet of rock and water, the water in the aquifer may be under pressure. So, when a well borehole punctures through that confining bed, the water in the well may rise above the top of the aquifer; this is called an artesian well. In some cases, the aquifer is under enough pressure that water will jet above the surface of the ground; this is called a flowing artesian well.
The process for determining flow direction is roughly the same, however. Based on the different elevations of the water in the wells, hydrologists map out a sort of imaginary water table above the surface of the aquifer called a potentiometric or piezometric surface. The shape of this piezometric surface provides the same visual that the water table does, though the piezometric surface is a measurement not of where groundwater is, but rather of how much pressure the groundwater is under. Groundwater will flow to the lowest point in the piezometric surface, which is the point of lowest pressure, or, more precisely, the point of lowest hydraulic head.
​
Why Does Flow Matter?
There is one key lesson to take from this: groundwater is a part of the water system, and it does move. As such, we need to think about it, even if we can’t see it. One obvious impact of groundwater’s movement is on stream flow: because groundwater moves so slowly, spring rains may not arrive to a stream or river until late summer, keeping water levels high even during dry spells. Groundwater can also carry contaminants meaning that a well “downstream” from a source of pollution can be contaminated just like a downstream stretch of a river. In that case, being able to calculate rate and direction of groundwater flow is extremely important. Finally, just as human intervention can obviously change surface water flow, pumping groundwater or creating impervious surfaces that prevent recharge can impact groundwater flow—and thus the movement of water in the entire system.
We can’t see it, but our understanding of and ability to measure groundwater is increasing rapidly; as such we must take this knowledge into consideration when making decisions about water resources. The remainder of the blog series (with an installment to be published every other week) will explore the some of these water resource decisions in more detail with a continued focus on the science behind them.
Surface Water Levels
A healthy water system with good groundwater recharge moderates water levels for surface water bodies like rivers and lakes. Our reputation as the Land of 10,000 Lakes is thanks to some pretty awesome groundwater, and we can’t forget that. The reason groundwater is so critical to keeping rivers flowing and lake levels up, even when there is no rain, is that it moves so slowly.
Spring is naturally a time for groundwater recharge in Minnesota because there is significant rain and snowmelt, but fewer plants and cooler temperatures mean that evapotranspiration is down. So extra water soaks into the ground, joins the groundwater, and begins the slow
journey “downhill” to the nearest surface water body. However, because groundwater travels so slowly, this may take weeks or months, so the spring rains that travel via groundwater arrive at their destination long after the rainfall. Thus, in summer, when hot temperatures and dry spells may stress water bodies, spring rain is still flowing into lakes, rivers, and streams. In some cases, this protects habitat for trout and other aquatic life by keeping streams cool and deep, even in summer. It can also just make a water body better for recreation by keeping water levels high enough for canoeing, boating, or swimming in hot summer months.
When groundwater recharge is interrupted, either by artificial drainage or impervious surfaces brought by urbanization, and run-off at the time of rainfall increases, the result is highly fluctuating water levels instead of a natural moderation of flow.
​
In Provinces 1 and 4 in Central Minnesota, where the surficial deposits are sandy, groundwater recharge is easy. In Provinces 2 and 5, with surficial clay that tends to infiltrate precipitation much more slowly, it becomes even more important to conserve soil structure either by practicing soil-friendly agricultural practices or by planting deep-rooted native plant species. Both of these practices create channels in the clay that allow water to infiltrate this material despite its low permeability. In Province 3, the exposed stripes of permeable bedrock tend to readily recharge groundwater and carry it to deep bedrock aquifers.
Contamination
While places that readily absorb groundwater recharge may seem to be the best place to be, there’s a flip side to this coin. Those same places are the places where contamination spreads the fastest. Since 75% of Minnesotans rely on groundwater as their drinking water, the nature of the spread of groundwater contamination is extremely important.
In some ways, water traveling through an aquifer is naturally filtered, either through the natural breakdown of contaminants over time, or because pollution gets caught on the surface of the rock as the water moves through it. For this reason, groundwater has often supplied safe drinking water
with little need for treatment–one of the reasons that, as the Twin Cities metro area population has expanded, the use of groundwater as a source of drinking water has outstripped the use of surface water.
However, the efficacy of an aquifer in acting as a filter depends largely on how quickly the water moves through it. Fast-moving water carries contamination with it, allowing for less time for chemical break-down. Furthermore, if water moves quickly the porosity of the rock is often higher, and just as a filter with larger holes catches less stuff, the rock with larger pores filters out fewer contaminants. So, in areas where groundwater seems pretty plentiful due to good recharge rates, the groundwater is most susceptible to contamination. We’re seeing the results of this now in Central and Southeast Minnesota (Provinces 4 and 3), where nitrate levels above the federal drinking water standard are forcing private well owners and municipalities to invest in expensive technology to make their groundwater drinkable.
​
Central and Southeast Minnesota may also be the canaries in the coal mine when it comes to groundwater contamination. Because groundwater moves slowly, we will not notice contamination in deep wells immediately, but once groundwater is contaminated, there is little we can do to address the problem in the ground. Even if the source of contamination is cut off, it can take years for the pollution to “work its way out of the system,” and nearby well owners may continue drawing up polluted water for quite some time.
​
When aquifers are confined, as is common in Provinces 1, 2, and 3, a confining layer can prevent or significantly slow the spread of contamination in one direction or another. However, this just means that contamination will spread all the more readily to another water user tapping the same confined aquifer.
​
Availability
It’s also important to remember that while some parts of the state have plentiful groundwater supplies, others are running dry. Even in the Twin Cities, which, based on the characteristics of groundwater Province 1, should have plenty of groundwater, the low levels of White Bear Lake indicate that this might not be the case. However, rural reaches of the state with much lower population density are feeling the stress as well. In Western Minnesota’s Province 5, the combination of bedrock with water available only locally in limited fissures and cracks and clayey surficial deposits that yield poorly make finding water difficult. Marshall, for example, is spending $13 million to develop a 27-mile pipeline to bring water to town. The smaller town of Mountain Lake has a similar plan: after several test wells closer to home turned up useless, the city plans to invest half a million in digging a new well farther away and piping the water in. And some towns haven’t been able to find water resources close to home at any cost—and instead are looking to import it from South Dakota via the Lewis and Clark Water Project.
Regardless of what issue seems most important in your region of the state, anyone who uses water needs to also be thinking about groundwater. Each of these challenges will require unique and thoughtful approaches to ensuring a sustainable supply of clean water.